The Auditory System: Perceiving Sound
- Joanne Lee
- Nov 24, 2020
- 5 min read
By Chris Jung
In my previous blog post I talked about the fascinatingly complex visual system and how it transmits electromagnetic energy and wavelengths into electrical energy processed by our neurons. Today, I’ll be talking about our auditory system: the physiological basis for how we hear. As with everything in neuroscience, the mechanisms behind these processes are endlessly complex, and I’ll just be going over some of the basic principles.
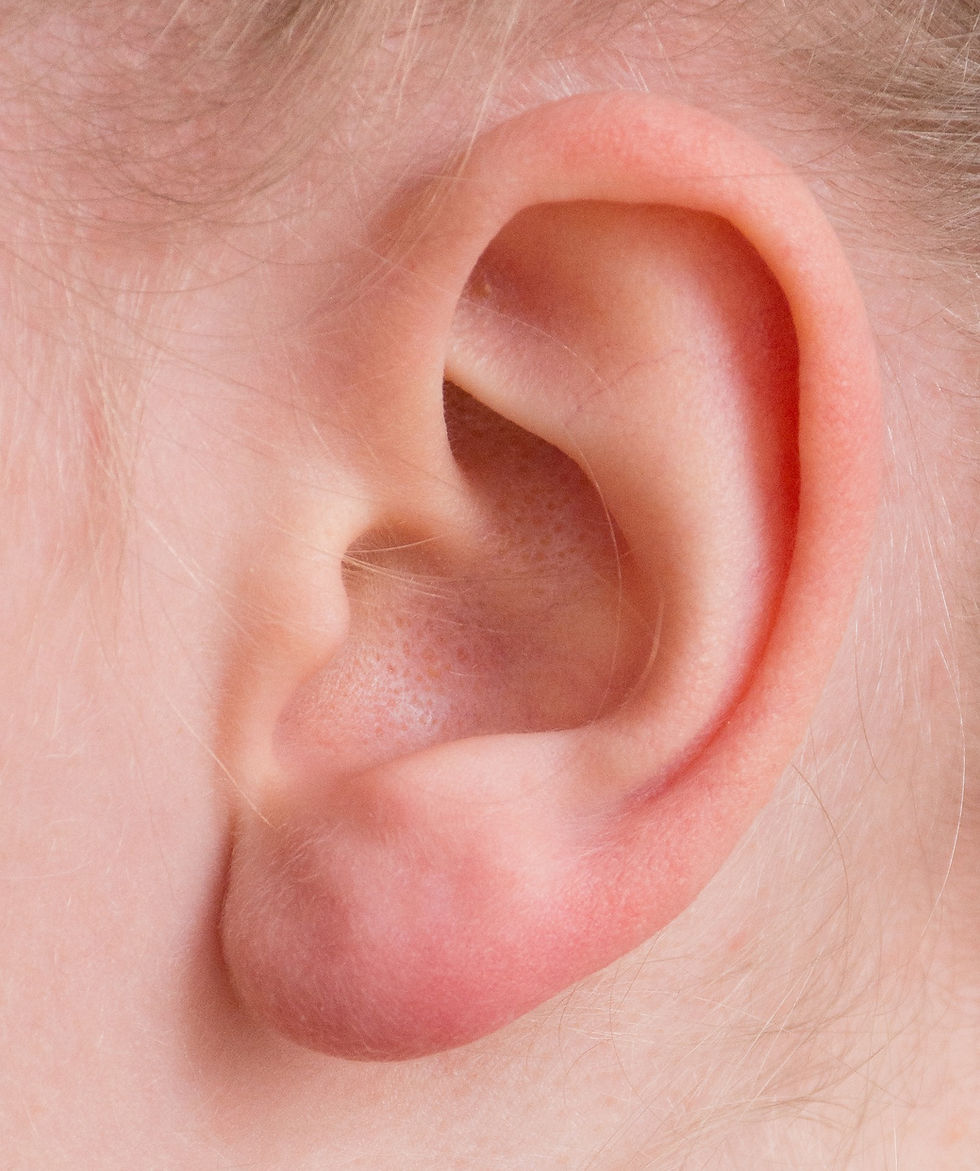
So how do we hear?
First, we’ll have to take it back to eighth grade science: what is sound? Well, the phenomena that we call “sound” results from the longitudinal waves in a medium such as air, where particles are forced into compressions and rarefactions, emitted at different frequencies (a bell will have more frequent compressions/rarefactions than the rumble of a motorcycle). Musical instruments can get a bit more confusing… the tiny, repetitive movements in hollow cylinders (flutes, clarinets) or the vibrations of strings (violins, pianos) are called standing waves because the ends of the material they pass through are fixed. Still, these standing waves generate longitudinal waves in the air around them.
But all of these examples deal with mechanical energy of particles moving… where do humans and our perception of this energy come into play?
These “pulses” of pressure” (compressions) are transmitted through the air to your ear canal (called your external auditory meatus). They then reach the tympanic membrane (or your eardrum) -- which separates your outer ear from your middle ear. The “pressure pulses” cause the tympanic membrane to vibrate. These vibrations are transmitted to three small bones (ossicles): the malleus, incus, then the stapes (latin for hammer, anvil, and stirrup, respectively). The stirrup is attached to the oval window (fenestra vestibuli), another membrane, which is also an opening to your cochlea.
Your cochlea is a snail-shaped spiral inner opening to your ear, which contains the Organ of Corti. It’s convenient to think of this organ as “the hearing portion” of your ear: like the photoreceptors in your eyes, the Organ of Corti is where energy is transformed into electrical neuronal information. This is accomplished because the vibrations from the oval window are transmitted throughout the membrane all the way to the tips of stereocilia in the hair cells of your cochlea (the cochlea is actually a hollow chamber of bone, where waves propagate from the base (the oval window) to the apex (the center of the spiral)). Stereocilia are like microscopic “hairs” that seem to “magically” act as sensors and activate neuronal information -- but we can have a better explanation than that!
The energy transduction process is thought to happen because the cochlea is filled with fluid that has a ton of electrolytes (namely calcium). When stereocilia move (due to the vibrations from sound waves), channels (receptors) open due to a cascade of protein conformations. Two membranes play a crucial role in this process: the basilar membrane and the tectorial membrane. The basilar membrane (BM) divides the fluid filled cochlea into two sections, the scala tympani and the scala vestibuli. The BM acts as the “floor” for the hair cells (which are connected to neurons). It also varies in thickness, being stiff and narrow at the base, allowing high frequency waves to propagate, and thicker at the apex, where low frequency waves would cause the entire membrane to vibrate. When the basilar membrane vibrates, the stereocilia of the hair cells brush against the tectorial membrane on top of them. The tectorial membrane acts as a “wall” that prevents the hair cells from fully moving; instead, the stereocilia are forced to bend, which causes the channels to open and sodium to depolarize the membrane. Consequently, the basilar membrane, hair cells, and tectorial membrane are together called the “Organ of Corti,” the hearing portion of the ear.
This electric depolarization from the hair cells travels through the vestibulocochlear nerve (which is the eighth cranial nerve). This nerve is composed of two neurons: the cochlear nerve and the vestibular nerve. The cochlear nerve is associated with the auditory system: the electrical impulse travels through its axons to the cochlear nuclei, the brainstem, and the primary auditory cortex within the temporal lobe. The vestibular nerve is more related to motion and position. It utilizes information from the “vestibular apparatus,” which is composed of the semicircular canals and two “otolithic organs” called the utricle and the saccule. (“Otolith” literally means “hearing stone,” because the stereocilia seem to be attached to stone-like structures). Although we won’t go into much detail about them here, there is extensive research that suggests the perpendicular orientation of the hair cells of these organs sense gravity and acceleration. Animals without otolith organs have trouble sensing motion as well as position relative to the ground, and have very poor posture. The vestibular nerve have axons that branch out to all these structures (the superior vestibular nerve is connected to the utricle, anterior, and lateral canals, and the inferior is connected to the saccule and posterior canal) and travels through the internal auditory meatus to the pons, and up to the vestibular nucleus in the medulla, where it branches extensively. Some of these branches reach the flocculonodular lobe in the cerebellum (more specifically, the fastigial nucleus), the spinal cord where it controls all sorts of muscle movements, to several other cranial nerves, and up to the ventroposterior medial nucleus in the thalamus where the signal travels through the corona radiata to the lateral post central gyrus, the insula, and the temporoparietal cortex!
These are a lot of structures and locations - and I haven’t even covered them all! The main take-away here is that the vestibular nerve and cochlear nerve join to form the eighth cranial nerve, but serve different functions and remain relatively separated in their deeper cortex pathways.
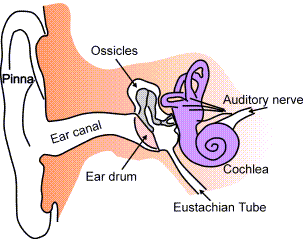
Our vestibular pathway is very important for movement and spatial awareness. Who would’ve thought that these functions would be so closely connected to our ability to hear!
Some other important structures in our auditory system are the round window and the Eustachian tube. The round window is an opening in our cochlea covered by the round window membrane, also called the second tympanic membrane. This allows the fluid in the cochlea to vibrate along with the basilar membrane, as the round window membrane can stretch out and cave in with more freedom than bone. In some congenital abnormalities, the round window is too rigid and the stapes cannot push fluid easily, resulting in hearing loss of about 60dB.
The Eustachian tube is a canal that branches out of the middle ear and connects it to the nasopharynx (upper throat and nasal cavity). It regulates the pressure in the middle ear, making sure it is equal to the air pressure outside the body. The tube remains closed except for when we yawn, swallow, or chew, or other situations where we may need to regulate pressure like being in an airplane or on a mountain. Defects in the eustachian tube usually involve only partial opening, which results in discomfort and “ringing” in the ear.
Consequently, inflammation due to allergies often plays a role in hearing/pressure problems.
In brief, mammalian hearing is usually the result of energy transformation from mechanical sound waves to electrical information caused by bending of the stereocilia on hair cells. The word “mammalian” should be taken lightly: dolphins and whales don’t have outer ears. Other animals like insects, reptiles, or birds may have sensors or “ear holes.” Anyways, I hope you learned something about hearing and perception!
Image Credit:
No changes were made to the following image, https://commons.wikimedia.org/wiki/File:Ear-anatomy-text-small-en.png, License: https://creativecommons.org/licenses/by-sa/3.0/legalcode
References/Further Readings:
Bordoni B, Mankowski NL, Daly DT. Neuroanatomy, Cranial Nerve 8 (Vestibulocochlear) [Updated 2020 Jun 11]. In: StatPearls [Internet]. Treasure Island (FL): StatPearls Publishing; 2020 Jan-. Available from: https://www.ncbi.nlm.nih.gov/books/NBK537359/
Straka, H., Zwergal, A., & Cullen, K. E. (2016). Vestibular animal models: contributions to understanding physiology and disease. Journal of neurology, 263 Suppl 1, S10–S23. https://doi.org/10.1007/s00415-015-7909-y
Vestibular system involvement in movement disorders and dizziness.
(2) Furman JM, Sparto PJ, Soso M, Marcus D. Vestibular function in migraine-related dizziness: a pilot study. J Vestib Res. 2005;15(5-6):327-332.
Good video for vestibular pathway neuroanatomy: https://www.youtube.com/watch?v=pxga9ci2ets
Comments