Selfish Genetic Elements
- Joanne Lee
- Aug 27, 2020
- 6 min read
By Jenny Lam
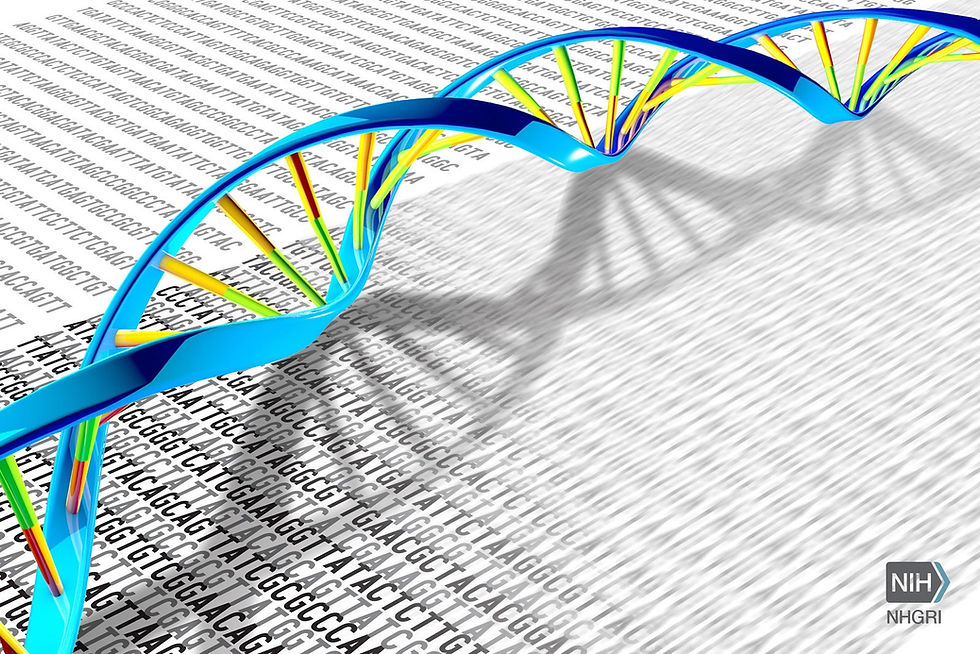
The genome is commonly viewed as a cohesive and singular unit that works to maximize an individual’s reproductive success. This concept stems from the fact that most harmful mutations are usually eliminated from the genome through natural selection, while beneficial traits that increase an individual’s fitness tend to persist and spread throughout the population. Because of this, the concept of conflicting genes has historically been a topic of controversy. However, a large portion of a eukaryote’s genome is composed of selfish genetic elements. Traditionally called “selfish” or “parasitic” genes, these are genetic units that can enhance their own transmission at the expense of other genes in the genome, but are either neutral or harmful to the individual carrying the gene. As a result of selfish genetic elements, conflicts arise among genes in the genome, called intragenomic conflict, which may be an important evolutionary force. The idea of selfish genetic elements was more widely considered after the popularization of a gene-centered view of evolution by Richard Dawkins in his famous book, The Selfish Gene. Dawkins proposed that natural selection is centered around the gene, rather than the individual and suggested that genes act to encode phenotypes that would increase their chances of transmission to the next generation even at the expense of the organism bearing the genes.
There are numerous types of selfish genetic elements, many of them having unique modes of transmission— but there are two universal ‘rules’ that apply to the majority of them. First, selfish genetic elements require sexual reproduction and outbreeding to spread. This is so selfish genetic elements can show up in new genetic lineages, whereas in asexual organisms, the selfish genetic elements are stuck in that lineage, and individuals without the harmful selfish genetic element are more likely to outcompete individuals with it. Secondly, the selfish genetic elements often reveal themselves in hybrid individuals through phenotypic consequences, as it is harder to identify their presence in nonhybrid populations. Many host genomes have evolved mechanisms to hinder the effects of selfish genetic elements, but it is more likely for a hybrid individual to inherit a certain selfish genetic element, but not inherit the mechanism to suppress its activity compared to its none-hybrid counterpart. Two popular examples of selfish genetic elements are transposable elements and meiotic drive.
Transposable elements, the most common type of selfish genetic element, are portions of DNA that can change their position in the genome of their hosts. Transposable elements are able to self-replicate and insert themselves throughout the genome through two methods: transposons use a “cut-and-paste” method, while retrotransposons, with the help of an RNA intermediate, propagate by the “copy-and-paste” method, leaving a copy of itself behind in the original location. These stretches of DNA are considered selfish genetic elements due to their ability to control their propagation in the genome and across hosts, behaving as if they were motivated by self-interests. Their insertions within different parts of the genome can disrupt important gene functions and cause a wide variety of harmful mutations in the hosts. Many animals and plants have developed mechanisms to reduce the effects of transposable elements by silencing them or interfering with their ability to move within the genome.
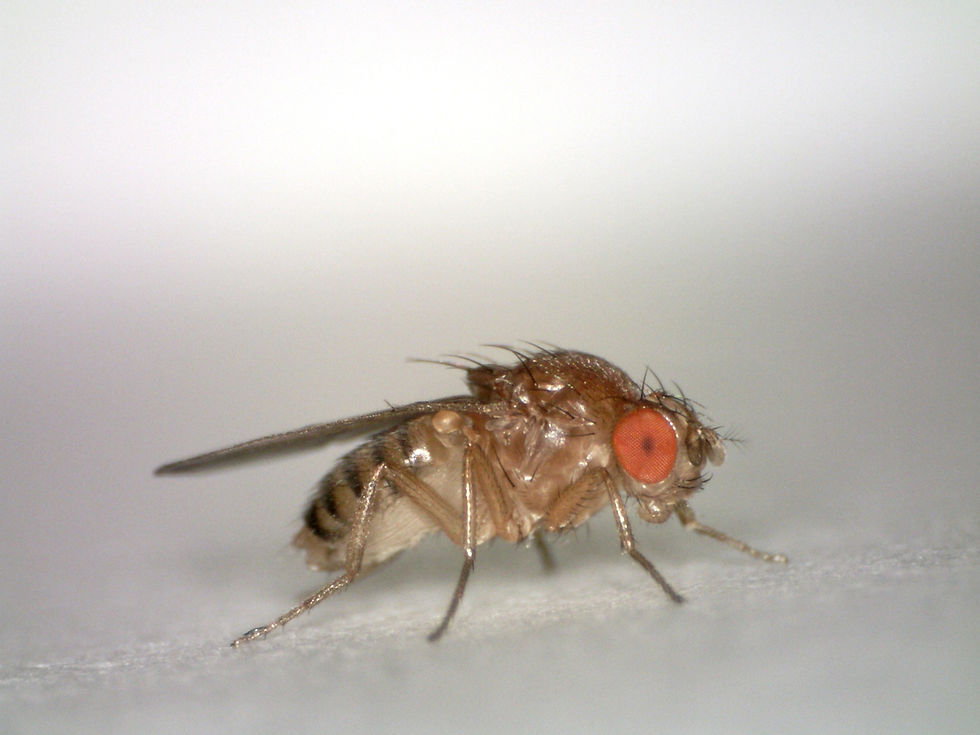
Another type of selfish genetic element is a meiotic drive, which refers to processes that cause certain alleles, called meiotic drivers, to be overrepresented in the gametes formed from meiosis. Meiotic drivers take advantage of the mechanisms of meiosis to increase their chances of transmission into gametes, often at the expense of the rest of the genome. In oogenesis, the production of female gametes, four products are made after the two rounds of meiosis, which includes 3 polar bodies and 1 egg. The polar bodies do not get passed to offspring and are reabsorbed instead. A meiotic driver that can increase its own chances at ending up in the egg rather than the polar bodies will increase its transmission rates and its frequency in the population. This can reduce an individual’s fitness if the meiotic driver is linked to harmful pleiotropic effects or mutations. The male meiotic drive can come in a variety of forms, all of which involve a driving element that prevents the function or maturation of sperm that does not contain the driver. Although this often reduces fertility, it increases the chances of passing on the drive gene to offspring. One of the best-described examples of this is Segregation Distorter in fruit flies (Drosophila melanogaster). Males that are heterozygous for the drive gene have the non-driver half of their spermatids made during spermatogenesis turn dysfunctional. Because males that are homozygous for the drive gene are sterile, the non-drive gene does not get eliminated from the population.
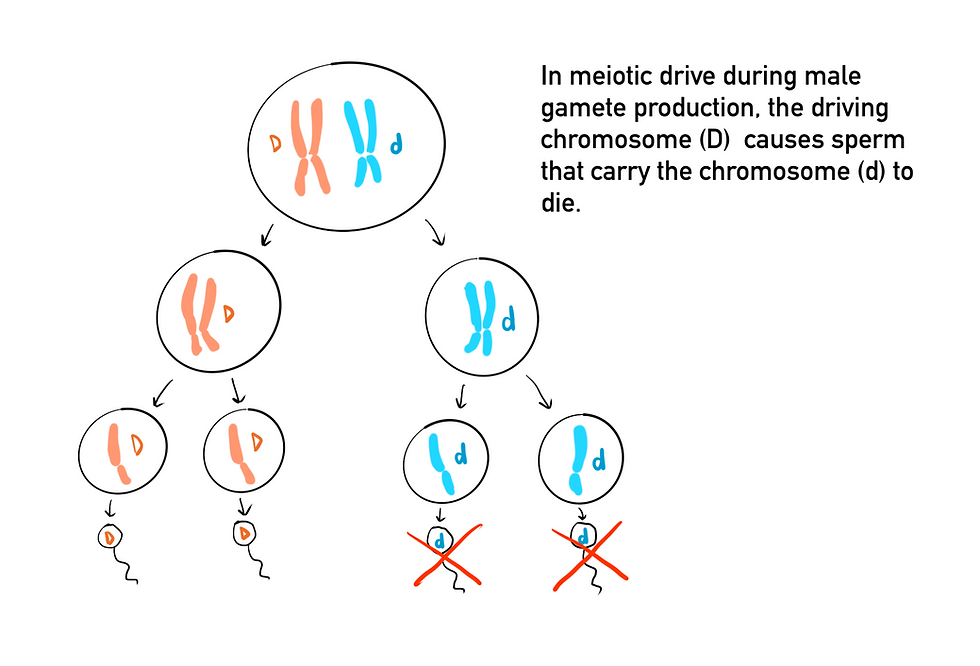
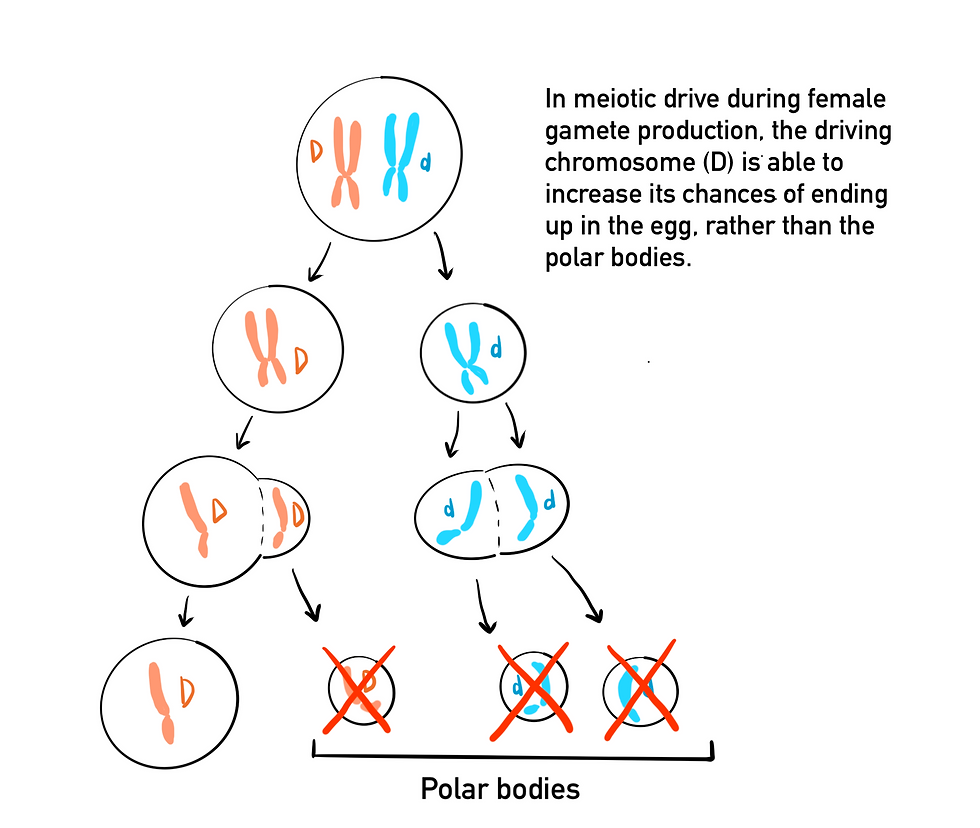
Selfish genetic elements can cause significant evolutionary effects, and without restriction, they can demonstrate that natural selection does not always prioritize organismal fitness. For example, in a situation where a selfish genetic element on the Y chromosome is able to direct sperm production such that males produce significantly more functional Y-bearing chromosomes than X-bearing chromosomes, the population would eventually have an extremely disproportionate male-based sex ratio. Thus, resulting in extinction when producing offspring becomes too challenging and inefficient. Selfish genetic elements, primarily B chromosomes and transposable elements, can contribute to genome size variation; through coevolution with their suppressors, selfish genetic elements can also lead to speciation. The flowering plant, Arabidopsis thaliana, has the same number of genes as that of Norway spruce, Picea abies, but the genome of Picea abies is around 100 larger due to the abundance of transposable elements. Although the idea of selfish genetic elements might seem counterintuitive from a Darwinian point of view when examining evolution at the level of the organism, these genetic units are far from fiction and their effects can be significant enough to impact the fate of many species.
What did you learn?
Questions:
1. What is the gene-centered view of evolution and how does it differ from the Darwinian viewpoint?
The gene-centered view of evolution frames evolution around the gene, suggesting that evolution occurs through the survival of competing genes as they “compete” to increase their frequency in the next generation, sometimes even at the expense of the organism. Genes do not actually compete with each other, as they are not creatures with goals and self-interests, so claiming that genes compete with each other or that they are trying to increase their frequency is simply a metaphor to explain that the gene is the unit of natural selection, according to this viewpoint. Supporters of this viewpoint argue that it can explain phenomena such as altruism and intragenomic conflict, which can decrease an organism’s fitness, rather than enhance it. It differs from a Darwinian perspective of evolution because, in the gene’s-eye view, the gene is regarded as the unit of selection, rather than the organism itself.
2. How do transposable elements move around the genome?
Transposable elements are able to change positions in the genome through several mechanisms. They can move using replicative methods (leaving a copy of themselves behind at the original location) or nonreplicative methods. Transposons, a type of transposable element, move within the genome using a DNA intermediate. In the “cut and paste” method, transposons make transposase, which cuts the element from the original site and moves it to a new site. This is nonreplicative because the DNA does not remain in its original location. Retrotransposons move using an RNA intermediate that is a transcript of the retrotransposon DNA using the “copy and paste method”. To travel to another position in the genome, the RNA intermediate is converted back into DNA by reverse transcriptase, which is inserted into another location. This leaves a copy of the original DNA at the initial site, as well as a copy of it at the new site.
Sources:
Ågren, J Arvid, and Andrew G Clark. “Selfish genetic elements.” PLoS genetics, vol. 14, 11 e1007700, 15 Nov. 2018, doi:10.1371/journal.pgen.1007700. Accessed 20 Aug. 2020.
Hurst, Gregory D. D., and John H. Werren. “The Role of Selfish Genetic Elements in Eukaryotic Evolution.” Nature Reviews Genetics, vol. 2, pp. 597-606, Aug. 2011, http://www.sas.rochester.edu/bio/labs/WerrenLab/My%20Papers/2001_Hurst&Werren.pdf. Accessed 20 Aug. 2020.
Hurst, Laurence D. “Selfish genes and meiotic drive.” Nature, 15 Jan. 1998, doi:10.1038/34526. Accessed 20 Aug. 2020.
Lindholm, Anna K, et al. “The Ecology and Evolutionary Dynamics of Meiotic Drive.” Trends in Ecology & Evolution, vol. 21, pp. 315-326, Apr. 2016, doi:10.1016/j.tree.2016.02.001. Accessed 20 Aug. 2020.
Werren, John H. “Selfish genetic elements, genetic conflict, and evolutionary innovation.” Proceedings of the National Academy of Sciences, 28 Jun. 2011, doi:10.1073/pnas.1102343108. Accessed 20 Aug. 2020.
Image Credit:
Comments