By: William Kang
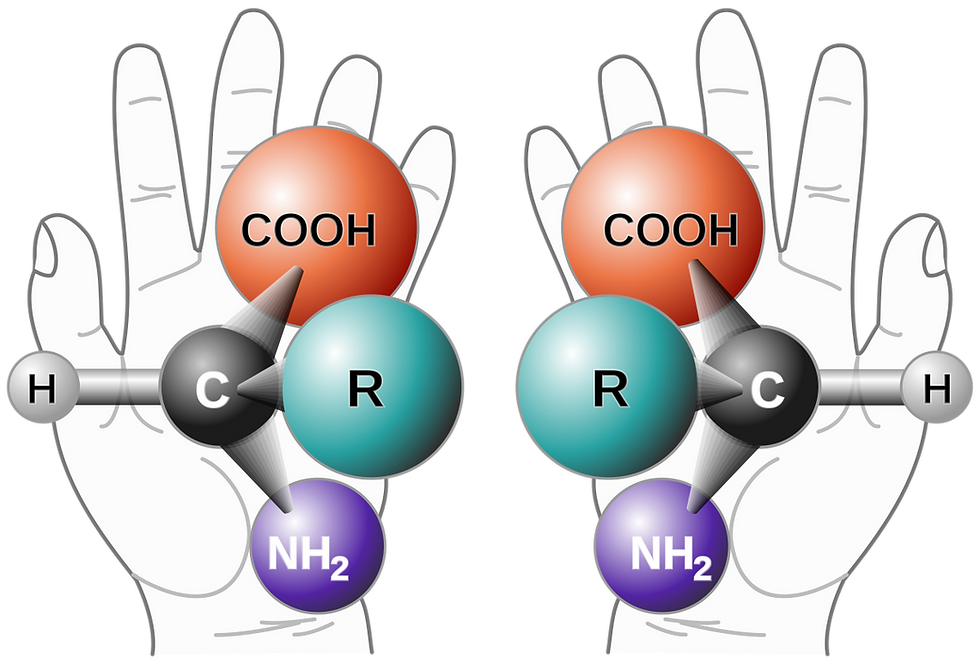
The word “chiral” comes from the Greek word for hand because hands are analogous to chiral molecules. If you face your hands in the same direction, it is clear that one hand cannot be superimposed onto the other. While your two hands look and are intended to function in essentially the same way, it is much harder to use your non-dominant hand than your dominant hand. In biochemistry, the term “chiral” is used to describe molecules inside of organic compounds, meaning they have a carbon atom at the center. Specifically, a chiral molecule is any molecule that cannot be rotated or translated to be identical with its mirror image. Analogous to hands, chiral molecules and their enantiomers (the mirror images) have the same chemical and physical properties, but can have drastically different biological/molecular interactions.
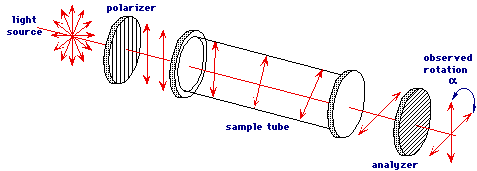
One way to distinguish between a chiral molecule and its enantiomer is by their optical activity. Chiral molecules are optically active, which means that when plane polarized light (light in which the electric field oscillates in just one plane) passes through a chiral molecule, an interaction will occur that causes the light to rotate by a certain degree. Because a chiral molecule and its enantiomer are mirror images, it follows that one enantiomer rotates the light by the same degree but in the opposite direction compared to the other enantiomer (clockwise vs counter-clockwise). If the light is rotated in a clockwise direction, the enantiomer is called dextrorotatory (+), and if the light is rotated in a counter-clockwise direction, the enantiomer is called levorotatory (-).
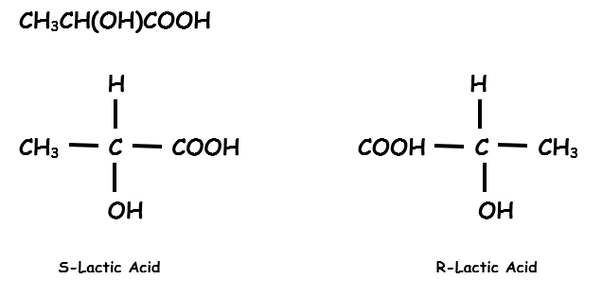
Chiral molecules are very important because many essential biological molecules are chiral, such as DNA and sugars. Due to the nature of chiral molecules, many of these biological molecules can only be formed by one enantiomer. In fact, using the other enantiomer in the biological molecule can have drastically different effects. For example, in the case of Thalidomide, a drug used as a sedative, it was found that using one enantiomer created the sedative (R-Thalidomide) while using the other enantiomer formed a potent teratogen (S-Thalidomide), which caused severe birth defects. This is because the spatial arrangement of the enantiomers are not the same. If one enantiomer were to be flipped onto the other, they would not be perfectly aligned. Going back to the comparison between hands and chiral molecules, even if you were to align your two hands, the arrangement would be different because your hands would be pointing in opposite directions. This property arises from the lack of a plane of symmetry in a chiral molecule, meaning that it is impossible to divide a chiral molecule into two equal parts.
Because using two enantiomers of a chiral molecule can have drastically different effects, it is important to be able to produce each enantiomer in a pure form. The two main ways to produce enantiomerically pure compounds are resolution and asymmetric synthesis. Resolution is a process where the two enantiomers of a racemic mixture (equal amounts of each enantiomer) are separated. Lipase enzymes are particularly sought after for this process because they have high catalytic activity, high enantioselectivity, and broad substrate specificity. High catalytic activity is the property by which lipase enzymes act as catalysts and increase the rate of reaction substantially. High enantioselectivity means that the lipase is able to distinguish one enantiomer from the other. Broad substrate specificity refers to lipases’ ability to act upon a broad range of substrates (“A chemical entity whose conversion to a product or products is catalyzed by an enzyme”), which makes lipases useful in a wide range of applications. The second way to produce enantiomers is through asymmetric synthesis. In asymmetric synthesis, a chemical reaction occurs that forms a different amount of each enantiomer. Currently, resolution is used a lot more in industries compared to asymmetric synthesis because it is cheaper and the ratio between the two pure enantiomers produced is consistent.

An important application of chiral molecules is in drug development. Over 50% of all current drugs and about 80% of drugs being currently developed are chiral drugs. However, the enantiomers of a chiral drug interact differently with proteins, enzymes, and receptors, so it is important to have the correct amount of each type of enantiomer. One way to visualize why only certain enantiomers function as intended is through Easson-Stedman’s illustration of a three point interaction between a drug and the receptor site. If three of the substituents of the enantiomer are labeled “A”, “B”, and “C”, and the corresponding receptors are labelled “a”, “b”, and “c”, then each substituent must be able to interact with the corresponding receptor for the enantiomer to be considered “active”. If one enantiomer is able to have each substituent interact with its corresponding receptor, then it follows that the other enantiomer cannot, by definition. The other enantiomer would be reflected, so “C” would match with “a”, “B” would match with “b” and “A” would match with “c”. Even if rotated, there is no way for the other enantiomer to be able to match all the substituents to the corresponding receptors.
Questions:
1. How can two enantiomers be differentiated if the physical and chemical compositions are the same?
One way to differentiate between two enantiomers is through their spatial arrangement. If you face the two enantiomers in the same direction, they are unable to be superimposed on one another. Another way to differentiate between enantiomers is through their optical activity. When plane polarized light passes through two enantiomers, it will be rotated by the same degree but in opposite directions. Finally, enantiomers can be differentiated by their different biological interactions. Because the spatial arrangements of each enantiomer is different, they will interact with the same receptors, proteins, and enzymes in different ways.
2. How can each enantiomer be produced in a pure form?
The most prevalent way to produce enantiomerically pure compounds is by the process of resolution. In resolution, an enzyme is used to separate a racemic mixture, which is a mixture with equal amounts of both enantiomers. The advantage of resolution is that the exact ratio between the two enantiomers is known. The other main way to produce pure enantiomers is by asymmetric synthesis. In this chemical reaction, enantiomers are produced in varying ratios.
Citations:
Comments