A Complete Introduction to Biomolecules
- Joanne Lee
- Aug 14, 2021
- 9 min read
By: Eliana Zhang
Although the average human diet is normally composed of both plants and animals, vegetarians have no trouble living on a diet entirely made of plants. Disregarding other commonalities (such as depending on water), this may seem like a surface-level comparison between plants and animals. If we look deeper, it becomes clear that plants could replace animals in food because they have the same molecular building blocks. When we eat plants, we digest their large biomolecules into smaller molecules. Our bodies then use them to build our own types of biomolecules. Although life is certainly diverse and employs types of molecules that are unique to organisms, the same basic structures enable the function of any organism’s cells.

The variation of biomolecules begins with the chemistry of carbon, the 6th element on the periodic table. It contains six protons, six neutrons, and six electrons, four of which buzz around in its outer shell. Since carbon needs eight electrons in its outer shell to be complete, it almost always shares electrons with the other elements to form an organic molecule: those such as carbon, hydrogen, nitrogen, oxygen, phosphorus, and sulfur. This four-electron shortage means a carbon atom can share with as many as four other elements. Its shape also results in stable and strong carbon-carbon bonds, which lead to long carbon chains that often appear in cells. Carbon is the essential atom for all organic molecules; the variety of life comes from when different atoms are added to a stable carbon structure.

Now that the carbon skeleton of a biomolecule has been established, it’s time to examine the combination of atoms that are attached to it. These are what differentiates biomolecules. Functional groups -- specific combinations of atoms that always react the same way -- largely influence the reactivity and function of a biomolecule. For instance, a hydroxyl group is made of an oxygen and a hydrogen (--OH). Adding a hydroxyl group to a molecule makes it an alcohol. If a hydroxyl group replaces one of a hydrogens in ethane, it becomes ethanol, a type of consumable alcohol. Hydroxyl groups are also polar, which means that electrons are not evenly distributed amongst its atoms. The addition of a hydroxyl group to ethane would turn it from a hydrophobic hydrocarbon to a hydrophilic alcohol. The ability to be soluble in water profoundly affects the function of biomolecules because cells are 70-90% water. While the carbon skeleton may help a biomolecule form its structure, functional groups like hydroxyl, carboxyl, and amine groups are what determine the biomolecule’s properties.
The largest biomolecules are polymers, which are made of identical subunits called monomers A protein, for example, can contain hundreds of amino acids. Most polymers are synthesized in the same way; a cell uses a dehydration reaction to join two subunits together by removing a water molecule. Two subunits generally have a hydroxyl group in one subunit, which has an oxygen and a hydrogen atom, and a hydrogen atom from another subunit. Removing these atoms results in a bond between the two subunits. To break down a biomolecule like how we digest plant cells to gain substance, cells use a hydrolysis reaction, which adds the water molecule back to where it was previously removed (“hydro” meaning water, “lysis” meaning destruction). A hydroxyl group from water bonds with one subunit while the remaining hydrogen atom attaches to the other. Both of these reactions only occur in the presence of enzymes, which are proteins that help bring the reactants together. The reaction would otherwise be too inefficient. An enzyme is a protein -- one of the four categories of biomolecules.
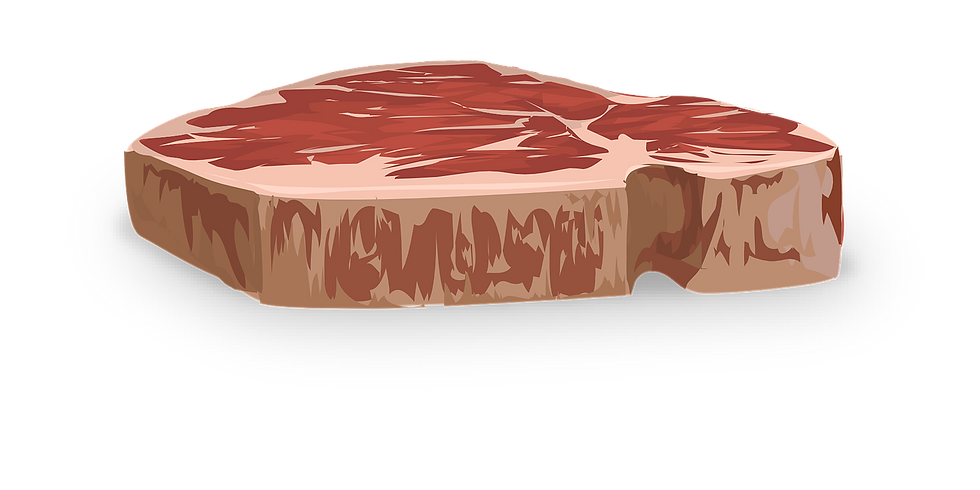
Proteins are polymers of amino acids. Amino acids have the same general structure; a central carbon atom is attached to a hydrogen atom, two functional groups, and a side chain called the R group. They’re called amino acids because the two functional groups are an --NH2 (amino group) and the other is a --COOH (an acid group). The R groups range from a single hydrogen atom to a complicated ring compound. Like all functional groups, some R groups are polar and others aren’t. The sequence of individual amino acids determines the shape of a protein, which then determines its structure and function.
Proteins are the most important biomolecule to each cell and have a wide array of functions:
Structure, such as in the silk protein in spider webs and the keratin protein in hair and fingernails.
Metabolism, such as enzymes, which are each specific to one type of reaction in a body.
Transportation, such as channel and carrier proteins in the plasma membrane.
Defense, such as antibodies that combine with invading viruses and bacteria to prevent them from destroying cells.
Regulation, such as the hormones insulin and glucagon, which regulate blood sugar.
Motion, such as proteins like actin and myosin, which cause muscles to contract.
Proteins are so integral to organisms that tissues are sometimes characterized by the proteins they contain. While we mostly get our protein from animal meat, plants like beans and nuts are also high in protein.
A cell cannot function with only proteins, though. Most cells have a plasma membrane that is essential to their structure because it separates the cell from its environment. Most of this membrane is made of phospholipids, which are part of another category of biomolecules called lipids. Most lipids are completely nonpolar and are thus insoluble in water. Phospholipids, however, are an exception -- they have a polar phosphate group that makes them soluble in water. These “polar heads” project outward and their nonpolar tails project inward. Two layers of these phospholipids thus form a “bilayer” in a watery environment.
Other lipids, called steroids, have hormonal functions. They have an entirely different structure. A steroid molecule has a carbon skeleton of four bonded carbon rings. One common steroid is cholesterol, which stabilizes an animal cell’s plasma membrane. The sex hormones testosterone and estrogen are also steroids. Testosterone can be responsible for increased muscular development, so athletes sometimes take anabolic, testosterone-enhancing steroids to improve their performance. This use, however, is mostly banned because steroid abuse can create health issues in the kidneys and cardiovascular system.
The second type of lipid is called a wax. They are solid at room temperature and hydrophobic, so they’re waterproof and can coat products like shoes to make water slide off of them. This use is seen in nature too. Waxes form a protective coating on plants to prevent loss of water. In animals, wax is produced to maintain skin and fur. For example, human ear canal glands produce earwax that can trap dust and dirt and prevent them from reaching the eardrum. Another popular instance of naturally occurring wax is honeybee comb cells, where beeswax is used to help contain honey.
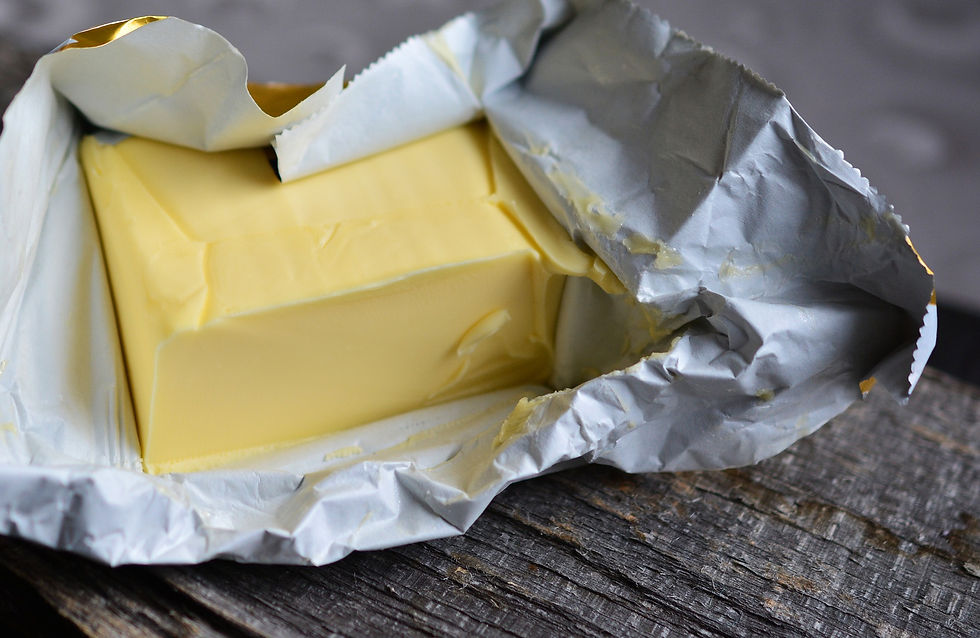
Fats and oils are more well-known lipids. They act as long-term, energy-storing molecules and contain two types of subunits: glycerols and fatty acids. Glycerol is made of three carbons each with a hydroxyl (--OH) group, and hydrogens form the rest of the bonds. A fatty acid has a long hydrocarbon chain with an acid group (--COOH) at an end. Fats and oils form when three fatty acids react with the carboxyl groups of one glycerol. Since there are three fatty acids attached to each glycerol molecule, they are called triglycerides. The exposed, polar functional groups of glycerol and fatty acid react with each other during this reaction, so triglycerides are nonpolar and hydrophobic. This is why fats and oils are insoluble in water. Fats like butter have saturated fatty acid chains, which mean there are no double bonds between carbon atoms and they are saturated with the maximum amount of hydrogen. Oils, on the other hand, have unsaturated fatty acids. They have double bonds between carbons, so there is not the maximum amount of hydrogen in the molecule. This double bond makes the fatty acid chain bend, which prevents close packing between the chains and enables the fluidity of oil. People often argue over the healthiness of saturated versus unsaturated fats, and the general consensus is that solid saturated fats are unhealthier. They can contribute to the buildup of abnormal lipids inside blood vessels, which is called plaque. Unsaturated oils protect against abnormal plaque buildup. Both saturated and unsaturated fats, however, can lead to obesity when consumed in large quantities. This may be a concern for people because the many carbon-hydrogen bonds present in lipids make them a richer source of energy, so it’s easy to over consume fats.
As a whole, lipids are the only biomolecules that are not polymers because they lack the repeated, identical monomers that characterize them . Entry biology textbooks may state otherwise, but at the heart of it, lipids are not organized by the standard polymer and monomer combination.
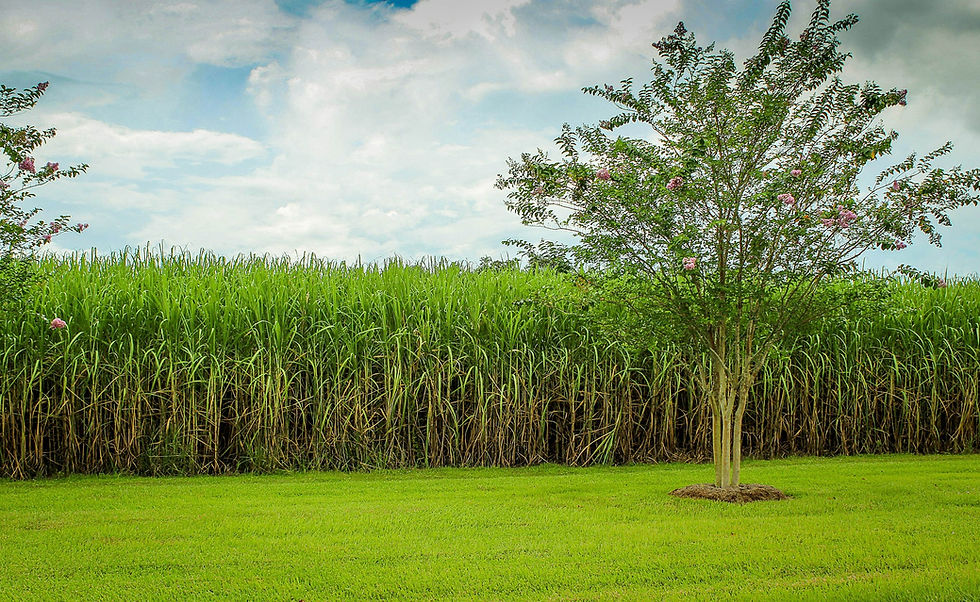
Carbohydrates are another good source of chemical energy. Some carbohydrates are long-term energy-storage molecules like fats, but others are a source of quick energy. Most carbohydrates have a carbon to hydrogen to oxygen ratio of 1:2:1 (CH2O) and are called that way because they are hydrates (H2O) of carbon (C). Simple carbohydrates -- monosaccharides -- are sweet sugars. Monosaccharides (“mono” meaning “one”, “sacchar” meaning sweet) are each composed of a single sugar molecule and are simple sugars that provide quick energy. Glucose is a common example. It is transported in the blood and is broken down for energy in nearly all types of cells. Ribose and deoxyribose are other monosaccharides, acting as 5-carbon sugars present in nucleic acid biomolecules. A disaccharide contains two monosaccharides joined together. Sucrose is a particularly important disaccharide because it is the transported sugar in plants, just as glucose is the transported sugar in animals. It is also the sugar we use for food, since it is present in plants like sugarcane and sugar beets, which are used to make common table sugar.
Complex carbohydrates are polymers with monosaccharide subunits. They are, therefore, polysaccharides (“poly” meaning multiple, “sacchar” meaning sweet). Some polysaccharides are short-term energy-storing molecules because they are much larger simple sugars. Their large size prevents them from passing through a cell’s plasma membrane. One example of a polysaccharide is starch in plants. Plants build starch with a dehydration reaction joining glucose monomers together, which removes a single molecule of water for every two molecules joined.
Starch can be “branched” or “unbranched”. When a polysaccharide branches, new polymer chains “branch off” at regular intervals. Unbranched polysaccharides lack those extra chains and are more simple. Both branched and unbranched forms act as glucose reservoirs in plants. Plant cells can complete the opposite of a dehydration reaction and add a molecule of water for every two molecules separated. This separates any two monomers joined by a dehydration reaction. Most importantly, plant cells use it to tap into starch reservoirs for energy. Animals convert plant starch to glucose with digestive enzymes.
Other types of polysaccharides are for structural purposes, such as cellulose, the most abundant carbohydrate and overall organic molecule on Earth. Cellulose’s subunit is glucose, but the majority of animals lack the enzymes to break down cellulose into subunits. Microorganisms in a mutualistic relationship with their host animal can break the bond between glucose subunits, like in termites and cows.

Carbohydrates also make an appearance in the final category of biomolecules: nucleic acids. Nucleic acids are divided into two types of polymers: DNA (deoxyribonucleic acid) and RNA (ribonucleic acid). The monomers of these are called nucleotides, which contain respectively the 5-carbon sugars deoxyribose or ribose The sugars in these nucleotides form part of the sugar-phosphate backbone that shapes a DNA or RNA strand. All nucleotides have a phosphate , a 5-carbon sugar, and a nitrogen base, -- called bases because they raise the pH of a solution. There are four nucleotides present in DNA and, on a given strand, they can appear in any order. Between the two strands of the DNA double helix structure, however, opposite “complementary” bases have to match : thymine is always paired with adenine, and guanine with cytosine. Thus, if you know the order of one strand, you can figure out the other. The bases in RNA are essentially the same except that thymine is replaced with uracil. When RNA is synthesized, RNA nucleotides pair with a DNA strand, so uracil pairs with adenine. There are many types of RNA in cells, but the one of the most significant is messenger RNA (mRNA) because it is a copy of a protein-synthesizing gene. After mRNA is made, it can move onto where proteins are made-- out of the nucleus. The mRNA acts as a “messenger” to let the rest of the cell know how to code for the specific sequence of amino acids in a protein. To summarize, genetic information about proteins goes from DNA to mRNA to the protein-making area.
The four kinds of biomolecules-- carbohydrates, fats, proteins, and nucleic acids-- are present in every cell and organism, but they are still so individually diverse and vary within each organism. You can get carbohydrates from both plants and animals, but while plants store glucose as starch, animals store it as glycogen. All biomolecules exist only because of the stable, lightweight, and versatile carbon atom. When looking at the world through the lens of science and biology, it is really a wonder that our vivid experiences are only made possible through Element Six on the periodic table.
What did you learn?
What are the four main categories of biomolecules?
Proteins, lipids, carbohydrates, and nucleic acids. Proteins are the most versatile biomolecule, and the shape of a protein is essential for its function. Lipids include fats and oils which are rich, energy-storing molecules. Other lipids are for structure, hormones, or protection. Simple carbohydrates are for quick energy, and complex carbohydrates store energy and provide structural support. Nucleic acids store genetic information.
What’s the difference between saturated and unsaturated fat?
Saturated fatty acid chains have maximized their number hydrogen bonds -- two hydrogens per carbon atom in the carbon chain. This allows for the chains to pack together tightly and solidify. Unsaturated fatty acid chains, however, have double bonds between carbons and have less than two hydrogens per carbon atom in a chain. This creates a bend and makes unsaturated fats liquid by preventing chains from packing together. Saturated fats are unhealthier because they contribute to plaque buildup in blood vessels, while unsaturated fats have been found to protect against that buildup.
Citations:
Image Credit:
Comments